Search For Similarity: Regulatory Considerations Of Drug Abuse Potential Investigations
By Anke Rosch, Boehringer Ingelheim
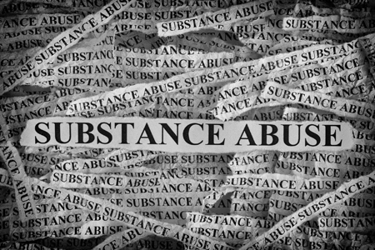
Human history seems inevitably associated with the phenomenon of substance abuse. This ranges from the ingestion of mind-altering substances such as Amanita muscaria (ibotenic acid and muscimol) in religious rituals in Central Asia and Siberia, to the extensive use of laudanum (opium) by the working class in the 19th century1 and abuse of new synthetic drugs permanently flooding the market. Compared to 2010, 275 million people took medications in 2019 (a 22% increase), with estimates of about 36 million people (+0.7%) suffering from substance use disorder.2 Opioid prescriptions increased by over 500%2 in the U.S. from 1997 to 2005. Overall, the death rate from opioid overdoses rose by almost six-fold3 between 1999 and 2017.
In 2000, the regulatory ball got rolling with a letter of the European Commission to the Committee for Proprietary Medicinal Products (CPMP) requesting a review of the potential for dependency/withdrawal reactions of selective serotonin reuptake inhibitors.4 In Europe this resulted in an EMA guidance on Non-clinical investigation of the dependence potential of medicinal products in 20065 and, in the U.S. FDA guidance for industry on the Assessment of Abuse Potential of Drugs in 2010,6 including clinical requirements in the latter. Both were preceded by the Scope of Application and the Guidelines for Animal Studies and Clinical Observations on Drug Dependence (1975, 1978)7, 8, 9 in Japan. In this article, I focus on EMA and FDA guidelines.
Definitions Of Drug Abuse & Abuse Potential Investigations
EMA and FDA guidelines begin with differences in the definitions of drug abuse/dependence. Drug abuse (FDA)6 or dependence syndrome (EMA)5 are defined in context with physical and/or psychological dependence based on the clinical diagnoses “substance dependence” or “substance use disorder” (ICD-1010 and 11,11 DSM 512).
After 1964,13 the stigmatizing diagnosis of addiction,14 which includes behavioral addictions (e.g., pathological gambling) in addition to substance dependence, was progressively replaced. A literature search in 2011 illustrates the difficulty of definition even within the concept of addiction in 2,994 peer-reviewed articles, of which 52 presented explicit statements that matched in only 20%.15
During drug development, abuse/dependence potential testing is mandatory for CNS active drug products/medicinal products that are novel (per both FDA and EMA) or that have a similar chemical structure and/or mechanism of action like those controlled under the U.S. Controlled Substance Act16 (CSA). The EMA guidance accepts limited testing for substances from classes known to cause dependence; no testing is an option for those that act similar to classes with documented absence of dependence. Metabolites need to be considered as well (EMA, FDA).
The FDA guideline presents several milestone investigations for the decision on the CNS activity of the drug throughout its life cycle, such as chemistry studies (e.g., drug structure/similarity), pharmacokinetic studies on distribution to and penetration of the brain, receptor/second messenger system binding studies, pharmacodynamic, and toxicological as well as clinical studies. Note 1 of the EMA guideline suggests defining a drug’s CNS activity by its entrance into the brain and interaction with central targets. Peripherally active drugs, e.g., abused at non-intended routes of administration, need consideration.
CNS Activity: Penetration Of The Blood-Brain Barrier
Drugs penetrate the blood-brain barrier (BBB) at high plasma concentrations, although sometimes this is unintended, as seen in animal toxicity studies,17 in patients with liver impairment or after misuse, e.g., due to drug tolerance. Loperamide (Imodium), for example, an over-the-counter antidiarrheal drug with agonistic activity at µ-opioid receptors, does not cross the BBB at therapeutic doses.18, 19 Co-administration with P‑gp and P450 3A4 inhibitors, however, may lead to euphoria overshadowed by the opioid overdose triad and cardiotoxicity. Based on a 91% increase in intentional loperamide exposures between 2010 and 2015, with 11 deaths,20 FDA released a safety alert post-marketing in 2016.21
Early investigation of BBB penetration during drug development is a basic need for lead optimization of CNS drugs. For peripherally active drugs, neither in- vitro nor in-vivo models are predictive enough for “non-CNS penetration”22 because of their lack of resolution and the incomplete understanding of brain permeation and distribution. The unbound brain-to-plasma partition coefficient Kp,uu,brain, describing the unbound drug concentration in the brain relative to blood at equilibrium,23 is characterized as a key driver for pharmacological responses. In a survey of 14 pharmaceutical companies,24 63% of the companies had implemented this concept into their project teams until 2015. About half of the companies (49%) tailored Kp,uu,brain measurements to individual drug discovery programs without establishing a default company-wide strategy.24 In early screening, 72% of survey responders collected first hints for BBB penetration and Kp,uu,brain extrapolation in transporter transfected cell lines (e.g., MDR1 and BCRP–Madin-Darby canine kidney cells),25,26 rather than in brain endothelial cells. All participants agreed on successful translation of in-vitro to in vivo results. Accepted in vivo methods are plasma and brain sampling with correction for plasma binding (up to 93% of participants) in PK and PD studies, PET/SPECT brain imaging (50%), or brain/blood microdialysis (14%).24
Quantitative whole-body autoradiography in rats in later development is often the first investigation of BBB penetration for peripherally active drugs. The brain/blood partitioning coefficient of all radiolabelled material quantifies metabolites and parent in brain tissue but also in its blood vessels.27 Discrepancies from Kp,uu,brain are the consequence.
Structural Similarity Screening
Although in the guidelines, chemical similarity screening to known substances of abuse is one decision criterion to determine whether a drug falls under their scope, no details are presented. A visual comparison of chemical structures is nearly impossible, taking the growing CSA list 16 of more than 543 substances into consideration. Computational chemistry investigations range from 2D substructure similarity analysis over 3D pharmacophore comparisons28 to prediction of biological targets and respective binding affinity.29 Remember that that the analysis is always retrospective and only involves structures/activities30 that were identified as abuse –related at the time of its performance. Thus, the results are strongly affected by substructure/target composition and their continuous updates on the analyzing software. This may lead to different outcomes of these investigations if performed in early development or later, e.g., before submission. Since country-specific regulations exist for controlled substances, the reference lists need to be carefully selected dependent on the countries where submission will be requested.
Investigation Of Pharmacological Similarity
The proof of pharmacological similarity to drugs of abuse and of potential CNS activity requires the characterization of its binding at respective brain targets. EMA touches these targets by citation of drug classes of concern. Targets were unequivocally specified by the FDA.
Neurobiologically, drugs act on the mammalian “reward” system. The mesocorticolimbic dopamine system involves pathways from the ventral tegmental area to the nucleus accumbens, it includes the amygdala and prefrontal cortex31,32 and is highly preserved across species.33 Originally, the system was designed for physiological reinforcers like food and sex, with a key role in maintenance and evolution of humanity.34 The FDA guideline relates the dopamine-, serotonin-, gamma-aminobutyric acid (GABA)-, opioid-, cannabinoid, and N-methyl-D-aspartate (NMDA) receptor systems, ion-channel complexes (e.g., calcium, potassium, chloride), and transporters (e.g., dopamine, serotonin, GABA) to abuse. However, the number of respective targets grows continuously in literature with time in parallel with the abuse receptor panels commercially offered. Usually, all drug candidates pass an in-vitro receptor panel35,36,37 at 10 µM as mandate by the FDA abuse guideline to detect potential off-target effects. The performance of an additional abuse potential target panel completing FDA requirements is often restricted to CNS drugs and ignored for peripherally active drugs. These assays only detect off-target binding affinity as percentage of binding, without characterization of functional activity (e.g., agonistic).
A follow-up testing of functional activity is recommended; however, a relevance threshold for percentage of binding is not defined by the guidelines. Papoian et al.38 issued a response of 50% as a criterion for positive response and functional testing. However, I cannot believe that the authorities will allow waiver of further abuse potential testing in cases of, e.g., 39% binding to an opioid receptor.
Conclusion
I consider an abuse potential assessment of novel drugs an absolute need before submission, although it does not necessarily result in dedicated studies when structural and/or pharmacological similarity to drugs of abuse could not be proven. It has gained importance due to the increasing numbers of drug addicts worldwide and the modification of substance use patterns to “in-house” available drugs during the COVID-19 pandemic.39 But the abuse potential guidelines do not define the start of respective activities since corresponding signals may come up during the entire drug development process.
The continuous growth in the number of abuse-related substances and brain targets may lead to repetition of front-loaded investigations later in development. Appearance of major metabolites from clinical studies40 triggers considerations for which studies must be supplemented, easily done for in-vitro tests but not for in vivo assays.
As illustrated for BBB investigations, the complexity of tests, the dependence of the results on test conditions and their impact on development times are challenging. Part 2 of this article series will discuss dedicated preclinical abuse potential tests in association with preceding studies. Particular emphasis will be placed on their predictivity for clinics based on the key question: How successfully can we model drug abuse in animal studies?
References
- Crocq MA. Historical and cultural aspects of man's relationship with addictive drugs. Dialogues Clin Neurosci. 2007;9(4):355-61. doi: 10.31887/DCNS.2007.9.4/macrocq. PMID: 18286796; PMCID: PMC3202501.
- United Nations, Office of Drugs and Crime. World Drug Report 2021.World Drug Report 2021 (unodc.org) (accessed on 16 September 2022).
- De Weerdt S. The natural history of an epidemic. Nature 2019; 573:10-12. d41586-019-02686-2.pdf (nature.com) accessed on 16 September 2022.
- EMEA/CPMP, European Medicine Agency, Committee for Proprietary Medicinal Products CPMP, 2000. Background to the CPMP Position paper on Selective Serotonin Uptake Inhibitors (SSRIs) and dependency/withdrawal reactions. EMEA/CPMP/2775/99. Background on CPMP Pos. paper on SSRIs and dependency-withdrawal reactions (europa.eu) (accessed on 16 September 2022).
- EMEA/CHMP, European Medicine Agency, Committee for Medicinal Products for Human Use, 2006. Guidance on the non-clinical Investigation of the Dependence Potential of Medicinal Products (https://www.ema.europa.eu/docs/en_GB/document_library/Scientific_guideline/2009/09/
WC500003360.pdf, (accessed 16 September 2022). - FDA/CDER, U.S. Department of Health and Human Services, Food and Drug Administration, Center for Drug Evaluation and Research, 2010. Guidance for Industry on the Assessment of Abuse Potential of Drugs, https://www.fda.gov/downloads/drugs/guidancecomplianceregulatoryinformation/guidances/
ucm198650.pdf (Accessed 16 September 2022). - Pharmaceuticals and Medical Devices Agency. Narcotics Division. March 14, 1975. Scope of Application and the Guidelines for Animal Studies and Clinical Observations on Drug Dependence, Notification No. 113 of the Narcotics Division.
- Pharmaceuticals and Medical Devices Agency. Narcotics Division. June 7, 1978, Guidelines for Animal Studies and Clinical Observations on Drug Dependence (Notification No. 383 of the Narcotics Division).
- Japan Pharmaceutical Manufacturers Association. Pharmaceutical administration and regulations in Japan. JPMA 2020; chapter 3: page 77. Pharmaceutical Administration and Regulations in Japan (individual chapters) | Pharmaceutical Regulations in Japan | Japan Pharmaceutical Manufacturers Association(JPMA) (accessed on 16 September 2022).
- World Health Organization (WHO). The ICD-10 classification of diseases and related health problems (10th revision). 1993. World Health Organization. Retrieved from ICD-10 Second Edition Volume 2 (who.int) (accessed on 16 September 2022).
- World Health Organization (WHO). ICD-11: International classification of diseases (11th revision). 2019 World Health Organisation Retrieved from ICD-11 (who.int) (accessed on 16 September 2022).
- American Psychiatric Association (Ed.). Diagnostic and statistical manual of mental disorders, 5th Edition: DSM-5. 5th ed. Arlington, VA: American Psychiatric Publishing; 2013. https://doi.org/10.1176/appi.books.9780890425596.
- Bykhovsky AF, Irlin IS. WHO Expert Committee on Addiction-Producing Drugs. 13th Report. World Health Organ Tech Rep Ser. 1964;273:1-20. PMID: 14127846.
- John F. Kelly, Richard Saitz & Sarah Wakeman (2016), “Language, Substance Use Disorders, and Policy: The Need to Reach Consensus on an ‘Addiction-ary’,” Alcoholism Treatment Quarterly, 34:1, 116-123, DOI: 10.1080/07347324.2016.1113103.
- Sussman S, Sussman AN. Considering the definition of addiction. Int J Environ Res Public Health. 2011 Oct;8(10):4025-38. doi: 10.3390/ijerph8104025. Epub 2011 Oct 20. PMID: 22073026; PMCID: PMC3210595.
- U.S. Department of Justice, Drug Enforcement Administration, Diversion Control Division, Drug & Chemical Evaluation section. June 2022. List of scheduling actions, controlled substances, regulated chemicals. Controlled Substances - Alphabetical Order - September 2022 (usdoj.gov) (accessed 05 October 2022).
- Eaton, D. L.; Klaassen, C. D. Principles of Toxicology. In Casarett & Doull’s Toxicology: The Basic Science of Poisons; Klaassen, C. D., Ed.; McGraw-Hill: New York, 2001; 6 ed.: 11-34.
- Passchier J, Comley R, Salinas C, Rabiner E, Gunn R, Cunningham VJ et al. The role of P-glycoprotein on blood brain barrier permeability of [C-11]Loperamide in humans. Neuroimage. 2008;41 (Suppl. 2):T192. https://doi.org/10.1016/j.neuroimage.2008.04.159.
- Kreisl WC, Liow JS, Kimura N, Seneca N, Zoghbi SS, Morse CL, Herscovitch P, Pike VW, Innis RB. P-glycoprotein function at the blood-brain barrier in humans can be quantified with the substrate radiotracer 11C-N-desmethyl-loperamide. J Nucl Med. 2010 Apr; 51(4):559-66. doi: 10.2967/jnumed.109.070151. Epub 2010 Mar 17. PMID: 20237038; PMCID: PMC2856602.
- Vakkalanka JP, Charlton NP, Holstege CP. Epidemiologic Trends in Loperamide Abuse and Misuse. Ann Emerg Med. 2017 Jan; 69(1):73-78. doi: 10.1016/j.annemergmed.2016.08.444. Epub 2016 Nov 4. PMID: 27823872.
- FDA. FDA Drug Safety Communication: FDA warns about serious heart problems with high doses of the antidiarrheal medicine loperamide (Imodium), including from abuse and misuse. Drug Safety and availability 2016. Retrieved from FDA Drug Safety Communication: FDA warns about serious heart problems with high doses of the antidiarrheal medicine loperamide (Imodium), including from abuse and misuse | FDA (accessed on 22 June 2022).
- Reichel A. The role of blood-brain barrier studies in the pharmaceutical industry. Curr Drug Metab. 2006 Feb;7(2):183-203. doi: 10.2174/138920006775541525. PMID: 16472107.
- Hammarlund-Udenaes M, Fridén M, Syvänen S, Gupta A. On the rate and extent of drug delivery to the brain. Pharm Res. 2008 Aug; 25(8):1737-50. doi: 10.1007/s11095-007-9502-2. Epub 2007 Dec 5. PMID: 18058202; PMCID: PMC2469271.
- Loryan I, Reichel A, Feng B, Bundgaard C, Shaffer C, Kalvass C, Bednarczyk D, Morrison D, Lesuisse D, Hoppe E, Terstappen GC, Fischer H, Di L, Colclough N, Summerfield S, Buckley ST, Maurer TS, Fridén M. Unbound Brain-to-Plasma Partition Coefficient, Kp,uu,brain-a Game Changing Parameter for CNS Drug Discovery and Development. Pharm Res. 2022 Apr 11. doi: 10.1007/s11095-022-03246-6. Epub ahead of print. PMID: 35411506.
- Wang Q, Rager JD, Weinstein K, Kardos PS, Dobson GL, Li J, Hidalgo IJ. Evaluation of the MDR-MDCK cell line as a permeability screen for the blood-brain barrier. Int J Pharm. 2005 Jan 20;288(2):349-59. doi: 10.1016/j.ijpharm.2004.10.007. Epub 2004 Dec 15. PMID: 15620875.
- Mahar Doan KM, Humphreys JE, Webster LO, Wring SA, Shampine LJ, Serabjit-Singh CJ, Adkison KK, Polli JW. Passive permeability and P-glycoprotein-mediated efflux differentiate central nervous system (CNS) and non-CNS marketed drugs. J Pharmacol Exp Ther. 2002 Dec;303(3):1029-37. doi: 10.1124/jpet.102.039255. PMID: 12438524.
- Solon EG, Balani SK, Lee FW. Whole-body autoradiography in drug discovery. Curr Drug Metab. 2002 Oct; 3(5):451-62. doi: 10.2174/1389200023337207. PMID: 12369892.
- Willett, P., Barnard, J.M., & Downs, G.M. Chemical Similarity Searching. J. Chem. Inf. Comput. Sci. 1998; 38: 983-996. DOI:10.1021/ci9800211.
- Ellis CR, Racz R, Kruhlak NL, Kim MT, Hawkins EG, Strauss DG, Stavitskaya L. Assessing the Structural and Pharmacological Similarity of Newly Identified Drugs of Abuse to Controlled Substances Using Public Health Assessment via Structural Evaluation. Clin Pharmacol Ther. 2019 Jul;106(1):116-122. doi: 10.1002/cpt.1418. Epub 2019 Apr 8. PMID: 30957872; PMCID: PMC6617983.
- Sheridan RP. Chemical similarity searches: when is complexity justified? Expert Opin Drug Discov. 2007 Apr; 2(4):423-30. doi: 10.1517/17460441.2.4.423. PMID: 23484752.
- Kelley AE, Berridge KC. The neuroscience of natural rewards: relevance to addictive drugs. J Neurosci. 2002 May 1; 22(9):3306-11. doi: 10.1523/JNEUROSCI.22-09-03306.2002. PMID: 11978804; PMCID: PMC6758373.
- Nestler EJ. Is there a common molecular pathway for addiction? Nat Neurosci. 2005 Nov; 8(11):1445-9. doi: 10.1038/nn1578. PMID: 16251986.
- Alcaro A, Huber R, Panksepp J. Behavioral functions of the mesolimbic dopaminergic system: an affective neuroethological perspective. Brain Res Rev. 2007 Dec;56(2):283-321. doi: 10.1016/j.brainresrev.2007.07.014. Epub 2007 Aug 21. PMID: 17905440; PMCID: PMC2238694.
- Nesse, R. M. (1994). An evolutionary perspective on substance abuse. Ethology & Sociobiology, 15(5-6), 339–348. https://doi.org/10.1016/0162-3095(94)90007-8.
- Whitebread S, Hamon J, Bojanic D, Urban L. Keynote review: in vitro safety pharmacology profiling: an essential tool for successful drug development. Drug Discov Today. 2005 Nov 1; 10(21):1421-33. doi: 10.1016/S1359-6446(05)03632-9. PMID: 16243262.
- Bowes J, Brown AJ, Hamon J, Jarolimek W, Sridhar A, Waldron G, Whitebread S. Reducing safety-related drug attrition: the use of in vitro pharmacological profiling. Nat Rev Drug Discov. 2012 Dec; 11(12):909-22. doi: 10.1038/nrd3845. PMID: 23197038.
- Lynch JJ 3rd, Van Vleet TR, Mittelstadt SW, Blomme EAG. Potential functional and pathological side effects related to off-target pharmacological activity. J Pharmacol Toxicol Methods. 2017 Sep;87:108-126. doi: 10.1016/j.vascn.2017.02.020. Epub 2017 Feb 16. PMID: 28216264.
- Papoian T, Chiu HJ, Elayan I, Jagadeesh G, Khan I, Laniyonu AA, Li CX, Saulnier M, Simpson N, Yang B. Secondary pharmacology data to assess potential off-target activity of new drugs: a regulatory perspective. Nat Rev Drug Discov. 2015 Apr; 14(4):294. doi: 10.1038/nrd3845-c1. Epub 2015 Mar 20. PMID: 25792260.
- Zaami S, Marinelli E, Varì MR. New Trends of Substance Abuse During COVID-19 Pandemic: An International Perspective. Front Psychiatry. 2020 Jul 16;11:700. doi: 10.3389/fpsyt.2020.00700. PMID: 32765328; PMCID: PMC7378810.
- FDA/CDER, U.S. Department of Health and Human Services, Food and Drug Administration, Center for Drug Evaluation and Research, 2020. Safety Testing of Drug Metabolites. Guidance for Industry. Safety Testing of Drug Metabolites Guidance for Industry (fda.gov) (accessed 16 September 2022).
About The Author:
Anke Rosch is a board-certified pharmacologist and toxicologist working at Boehringer Ingelheim Pharma GmbH & Co. KG. A doctor of veterinary medicine, she has more than 20 years of experience in the pharmaceutical industry and has special expertise in safety pharmacology. Anke can be reached at ankerosch.PharmacolTox@t-online.de.